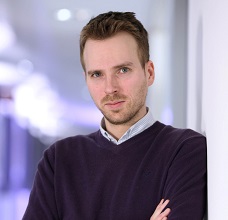
As a Research Fellow, we are assigned to short, inter-disciplinary collaborations known as “seed corn projects”. This scheme aims to help undeveloped seeds of ideas germinate and take shape as fully-fledged research programmes. Ironically, my first such project was to work on a fundamental problem of growth in developmental biology known as morphogenesis. Taken from Greek, this literally means “the beginning of the shape” and defines the process by which living organisms develop their shape, patterns and other biological structures (Figure 1). Given that many species begin as an embryo dividing into a uniform collection of genetically identical cells, it was a deep mystery how those cells could start to differentiate themselves into stripes, spots or organs. While this puzzle captured the imagination of story-tellers like Rudyard Kipling, it is not until we can quantitatively predict these patterns that we have a solid understanding of the process which generates them.

Cracking the developmental code
Taking a break from inventing the computer and breaking the Enigma cipher, the first attempt to explain this process mathematically was by the great Alan Turing in 1952 – just two years before his untimely and mysterious death. In his landmark paper, The Chemical Basis of Morphogenesis, he proposed an elegant model based on two chemicals known as morphogens (Figure 2). Rather than passively and evenly moving through the embryo, these molecules interact with each other as they diffuse, giving rise to different concentrations in different regions. The varying levels of the morphogens then act as chemical switches, affecting the cells differently and triggering the development of structure. Powerfully, the same model explains a huge array of the different forms observed in nature by tweaking only its handful of parameters.
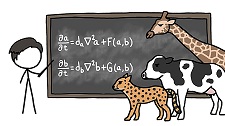
Touch and grow
While Turing’s ground-breaking work remains one of the founding studies in theoretical biology, as ever, nature is more messy and complicated. Using modern microscopes and experimental techniques, biologists have observed additional ways in which morphogens are transported throughout the embryo. One way is by placement with finger-like projections called cytonemes (Figure 3). Through cytonemes, cells can “reach out” to other specific cells, quickly and tidily depositing the morphogen they carry on their tips (think E.T.!). This active and targeted mechanism is central to the research on morphogenesis in the laboratory of Prof. Steffen Scholpp. In particular, it may explain why the borders between brain regions in the zebrafish appear so quickly and so neatly.
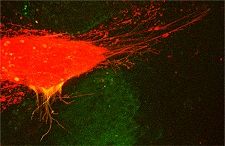
Bits, brains and growing pains!
To understand this process, I began to develop a model which could be simulated by computers (Figure 4). Models can be particularly useful in biology as, once properly fit to the data, they can take the place of difficult and time-consuming experiments. The predictions they generate can then guide experimenters to more effectively “hone-in” on interesting results as well as provide important insights into the underlying principles of the system. While software exists to simulate the Reaction-Diffusion equations described by Turing, none was available to model cytonemes. I therefore set about developing this simulation in a framework called Chaste. This proved to be a steep learning curve, so I initiated a collaboration and secondment with Dr. Alex Fletcher – one of the software’s developers. Having climbed the mountain to get Chaste working, I developed and published a Docker image to ease the pain of its wider community of users!
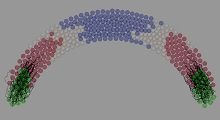
Science not Fiction
Now that we have a working model, the next step is to make it as close to the biological system as possible through further measurements and carefully setting the parameters. Once calibrated, we will begin the cycle of testing ideas with the model and then confirming (or refuting and refining) them with experiments. This would help to provide a clear understanding of how different factors contribute to the formation of the zebrafish’s brain (and morphogenesis in general). A better understanding of morphogenesis could lead to new possibilities for correcting developmental disorders, regenerating tissue, stopping cancer or even enhancing normal growth processes. This research may be less entertaining than How the Leopard Got His Spots but ultimately we hope, also more useful than a Just So story!